Caroline Laplante PhD
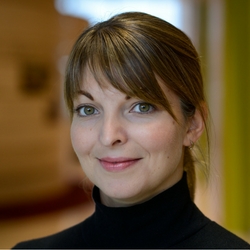
Bio
Caroline Laplante joined NC State in January 2017 as a Chancellor’s Faculty Excellence Program cluster hire in Modeling the Living Embryo. As an assistant professor in the Molecular Biomedical Sciences Department at the College of Veterinary Medicine, she will focus on uncovering the complex molecular organization and dynamics of biological contractile structures in single cells and in developing organisms. As a quantitative cell and developmental biologist, Laplante answers these fundamental questions using a combination of genetics, quantitative fluorescence microscopy and high-speed super-resolution imaging in live cells and tissues.
Laplante trained as a biochemist and obtained her Ph.D. in biology from McGill University. During her doctoral studies, she discovered an essential role for the differential expression of the cellular adhesion protein Echinoid in the polarized assembly of the contractile leading edge in the migrating dorsal epithelium during embryogenesis in Drosophila. Her postdoctoral work challenged commonly accepted notions about the cytokinetic contractile ring essential for cell division in animals, fungi and amoebas. She discovered that not one, but multiple myosins function to generate the forces necessary for cell division and characterized the distinct role of these three myosins during the assembly and constriction of the contractile ring in fission yeast. Laplante adapted high-speed Fluorescence PhotoActivated Light Microscopy (FPALM) to the study of live fission yeast cells. With this technique, she obtained quantitative images of the cytokinetic apparatus at unprecedented resolution. She discovered that cytokinetic protein assemblies called “nodes” are the basic unit of constriction of the contractile ring in fission yeast, and built a molecular model of the protein organization within the node.
Area(s) of Expertise
COMPUTATIONAL BIOLOGY AND BIOINFORMATICS
Our research applies precision measurements of molecular, cellular and tissue dynamics in living embryos, along with computer simulation and modeling, to understand the development, growth and diversification of plants and animals.
Publications
- The Myosin-V Myo51 and Alpha-Actinin Ain1p Cooperate during Contractile Ring Assembly and Disassembly in Fission Yeast Cytokinesis , JOURNAL OF FUNGI (2024)
- Actin-Microtubule Crosstalk Imparts Stiffness to the Contractile Ring in Fission Yeast , CELLS (2023)
- Microtubule competition and cell growth recenter the nucleus after anaphase in fission yeast , MOLECULAR BIOLOGY OF THE CELL (2023)
- Imp2p forms actin-dependent clusters and imparts stiffness to the contractile ring , MOLECULAR BIOLOGY OF THE CELL (2022)
- Laser ablation reveals the impact of Cdc15p on the stiffness of the contractile ring , MOLECULAR BIOLOGY OF THE CELL (2022)
- Host-Pathogen Interactions of Chlamydia trachomatis in Porcine Oviduct Epithelial Cells , PATHOGENS (2021)
- Molecular organization of cytokinesis node predicts the constriction rate of the contractile ring , JOURNAL OF CELL BIOLOGY (2021)
- Building the contractile ring from the ground up: a lesson in perseverance and scientific creativity , Biophysical Reviews (2018)
- Resolving single-actin filaments within the contractile ring of fission yeast , PROCEEDINGS OF THE NATIONAL ACADEMY OF SCIENCES OF THE UNITED STATES OF AMERICA (2018)
- Response to Zambon et al. , Current Biology (2017)
Grants
Cytokinesis, the separation of a mother cell into two daughter cells, is one of life������������������s most fundamental processes; it is central to the making of multicellular organisms and the transmission of genetic material across generations. The core machinery of cytokinesis is a contractile ring of actin, myosin motors and other proteins and is conserved from fungi to human. The contractile ring is connected to the inside of the cell cortex and its constriction leads the ingression of the plasma membrane into a furrow between the two cytoplasmic compartments that will become individual cells after the completion of cell division. Furrow ingression requires the cooperation between two main mechanisms: 1) constriction of actin filaments by the action of myosin motors and 2) the transmission of this contractile force to the plasma membrane via anchor proteins that connect the actomyosin bundle to the plasma membrane. We found that the proteins of the contractile ring occupy distinct layers with plasma membrane-interacting proteins in the outer layer adjacent to the cortex and the force-producing myosin motors in the inner layer. The next frontier in understanding the mechanism of cytokinesis is to determine how these proteins are organized into complex structures, how these structures move within the contractile ring and are removed from the ring during constriction, and how this dynamic architecture governs the force-generation function of the contractile rings. The objective of this application is to determine the anchoring role of the outer ring and the tension-force producing role of the inner ring by uncovering their dynamic architecture and effects on mechanics of constriction. Our hypothesis is that furrow ingression results from contractile forces produced in the inner layer of the contractile ring, conveyed to the plasma membrane via anchoring achieved by proteins in the outer layer. We plan to test our central hypothesis with the following Specific Aims: 1) determine how cytokinetic node proteins anchor the contractile ring and transmit contractile forces to the plasma membrane during furrow ingression and 2) determine how the molecular architecture of the inner ring governs the constriction of the contractile ring during furrow ingression. The proposed research in this application is innovative because we will use a unique combination of high-speed Fluorescence Photoactivation Localization Microscopy (hsFPALM) in live cells to determine protein organization and its dynamics with laser microsurgery to probe the mechanics of this tension-force producing machine in live cells. The proposed research in this application is significant as it will result in the identification of previously unknown parameters for new molecular and functional models of the contractile ring. As the proteins of the contractile ring are conserved from yeast to human, we expect that the core mechanism of cytokinesis elucidated in fission yeast will act as a template for understanding the mechanism of cytokinesis and even perhaps other non-muscle contractile cellular machines in other species.
There is substantial evidence that chromatin (genomic DNA and the proteins, RNAs, and chemical motifs bound to it) is a central regulator of diverse cellular and disease processes, in particular through its regulation of gene expression. Despite the wide-spread acceptance of its importance and relevance throughout biology, it is remarkable that our understanding of chromatin������������������s mechanisms and functions remains very limited and reliant on largely correlative observations or non-specific genome-wide perturbations. Here we propose the engineering of two synergistic and parallel sets of tools. One set of tools will sense and report on the dynamic biochemical and conformational states of chromatin in living mammalian cells. The other set of tools will dynamically induce changes in or ����������������edit��������������� chromatin biochemistry and conformation at specific user-defined genomic loci.
��������������� Aim 1: To determine the molecular organization and protein numbers within the nodes. This aim will be addressed by endogenously tagging representative node proteins with fluorescent markers and documenting the resulting localization patterns in live cells. High-speed Fluorescent Photo-Activated Light Microscopy (hsFPALM) will also be utilized in order to characterize the dynamic structures within the contractile ring and the cytokinetic nodes. ��������������� Aim 2: To determine how modulating the composition and number of nodes alters the mechanical properties of the contractile ring. This aim will be addressed by genetically modulating the number and composition of the cytokinetic node proteins using various genetic backgrounds, and quantifying those through the use of quantitative confocal microscopy. In order to measure the effect on the mechanical properties of the contractile ring, laser ablation will also be performed. In doing this, we will be able to evaluate the impact of node variation on the anchoring of the contractile ring. ��������������� Aim 3: To develop a force sensor to internally measure force production exerted by the contractile ring. Due to the lack of available tools, the force production inside of living cells is difficult to quantify. With a force sensor, the force exerted by the contractile ring can be more efficiently measured. We will design various biosensors based on the principle of Fluorescence Resonance Energy Transfer (FRET) in order to calculate the force exerted by the contractile ring during constriction, as well as the forces exerted between the contractile ring and the plasma membrane. Internal forces exerted between the main actin bundle and both the node proteins and the individual actin filaments will also be probed.
��������������� Aim 1: To determine the molecular organization and protein numbers within the nodes. This aim will be addressed by endogenously tagging representative node proteins with fluorescent markers and documenting the resulting localization patterns in live cells. High-speed Fluorescent Photo-Activated Light Microscopy (hsFPALM) will also be utilized in order to characterize the dynamic structures within the contractile ring and the cytokinetic nodes. ��������������� Aim 2: To determine how modulating the composition and number of nodes alters the mechanical properties of the contractile ring. This aim will be addressed by genetically modulating the number and composition of the cytokinetic node proteins using various genetic backgrounds, and quantifying those through the use of quantitative confocal microscopy. In order to measure the effect on the mechanical properties of the contractile ring, laser ablation will also be performed. In doing this, we will be able to evaluate the impact of node variation on the anchoring of the contractile ring. ��������������� Aim 3: To develop a force sensor to internally measure force production exerted by the contractile ring. Due to the lack of available tools, the force production inside of living cells is difficult to quantify. With a force sensor, the force exerted by the contractile ring can be more efficiently measured. We will design various biosensors based on the principle of Fluorescence Resonance Energy Transfer (FRET) in order to calculate the force exerted by the contractile ring during constriction, as well as the forces exerted between the contractile ring and the plasma membrane. Internal forces exerted between the main actin bundle and both the node proteins and the individual actin filaments will also be probed.