Kurt Marsden
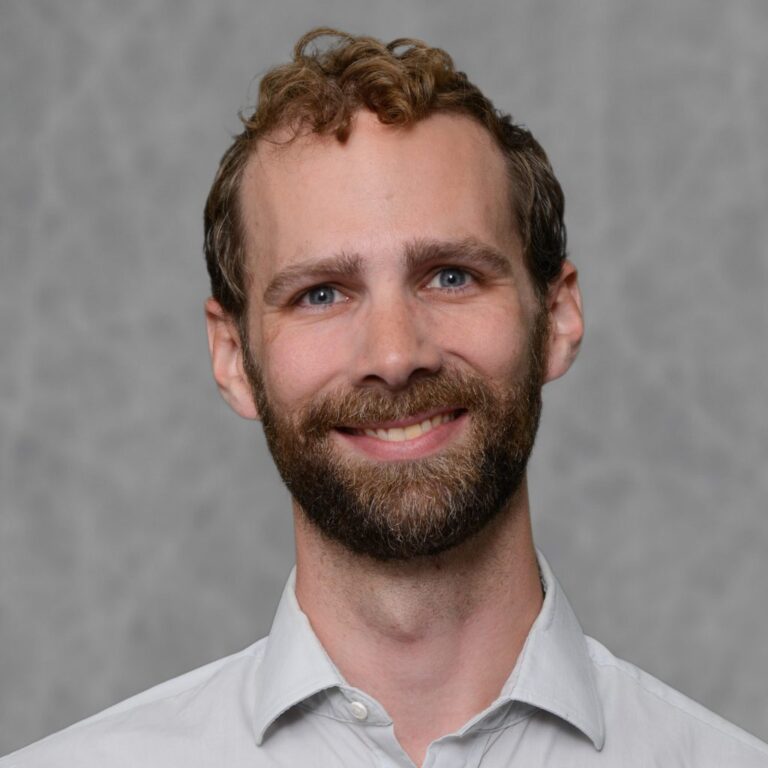
Bio
Dr. Kurt Marsden received his B.A. from Sarah Lawrence College, where he pursued a variety of subjects including music, history, psychology, and the natural sciences. After graduating, he entered the Medical Scientist Training Program (MSTP) at Albert Einstein College of Medicine, where he received his Ph.D in Neuroscience. His thesis work with Dr. Reed Carroll revealed novel molecular pathways underlying the plasticity of inhibitory synapses in the hippocampus and earned him the Julius Marmur Research Award. Dr. Marsden then did his postdoctoral research with Dr. Michael Granato at the University of Pennsylvania, studying the genetics of behavior in larval zebrafish.
Building off of this work, research in the Marsden lab is focused on understanding how genes and environmental factors guide the formation and function of neural circuits to drive behavior in zebrafish. Dr. Marsden is particularly interested in how information from the environment is translated by the brain into meaningful behavioral responses. His lab uses a variety of experimental approaches including high-throughput behavioral testing, 3D analysis of neural circuit morphology, simultaneous imaging of neuronal activity and behavior, and gene expression analysis. This work can help to reveal cellular and molecular pathways that underlie aspects of diseases such as autism and anxiety.
Publications
- Effects of 4 Testing Arena Sizes and 11 Types of Embryo Media on Sensorimotor Behaviors in Wild-Type and chd7 Mutant Zebrafish Larvae , ZEBRAFISH (2024)
- Developmental cadmium exposure disrupts zebrafish vestibular calcium channels interfering with otolith formation and inner ear function , NEUROTOXICOLOGY (2023)
- Effects of 4 testing arena sizes and 11 types of embryo media on sensorimotor behaviors in wild-type andchd7mutant zebrafish larvae , (2023)
- Morphological and sensorimotor phenotypes in a zebrafish CHARGE syndrome model are domain-dependent , GENES BRAIN AND BEHAVIOR (2023)
- Morphological and sensorimotor phenotypes in a zebrafish CHARGE syndrome model are domain-dependent , (2022)
- The Cyanotoxin 2,4-DAB Reduces Viability and Causes Behavioral and Molecular Dysfunctions Associated with Neurodegeneration in Larval Zebrafish , NEUROTOXICITY RESEARCH (2022)
- A forward genetic screen identifies Dolk as a regulator of startle magnitude through the potassium channel subunit Kv1.1 , PLOS GENETICS (2021)
- Electrical synaptic transmission requires a postsynaptic scaffolding protein , ELIFE (2021)
- Pioneer Axons Utilize a Dcc Signaling-Mediated Invasion Brake to Precisely Complete Their Pathfinding Odyssey , JOURNAL OF NEUROSCIENCE (2021)
- The cyanotoxin 2,4-DAB enhances mortality and causes behavioral and molecular dysfunctions associated with neurodegeneration in larval zebrafish , (2021)
Grants
A fundamental function of the nervous system is to distinguish between threatening and non-threatening stimuli. For example, a sudden intense sound that indicates danger should trigger an acoustic startle response, but an innocuous sound should not. This type of behavioral threshold is a basic mechanism for sensorimotor filtering, and the importance of setting this threshold appropriately is highlighted by the startle hypersensitivity observed in neuropsychiatric diseases such as autism, anxiety, and schizophrenia. Despite its importance, and in contrast to our knowledge of experience-dependent startle modulation, the molecular and cellular pathways that establish and maintain the innate startle threshold are not well characterized. By developing a more complete understanding of the biological mechanisms that govern the startle threshold, we can generate new hypotheses about the neural bases for these diseases. This project will leverage the powerful larval zebrafish model system to investigate the molecular-genetic and neural circuit bases of the startle threshold. Here a simple, conserved, and genetically accessible circuit drives a stereotyped startle response, with auditory afferents triggering reticulospinal neurons to activate motor neurons and initiate movement. In a recent genome-wide screen, we identified a novel regulator of the innate startle threshold: cytoplasmic Fragile X mental retardation protein (FMRP) interacting protein 2 (cyfip2). cyfip2 mutants are hypersensitive and startle to low intensity sounds that rarely startle wild-types. Cyfip2 acts through FMRP and eIF4E to regulate RNA translation, but it can also control actin polymerization through interactions with Rac1 and the WAVE regulatory complex (WRC). In Aim 1 we will systematically test which of these molecular pathways cyfip2 uses to establish the startle threshold and to maintain it through development. In Aim 2 we will define the cellular basis for cyfip2-mediated threshold control by first locating the site of the primary circuit defect with optogenetic and calcium imaging approaches and then identifying the cell types in which cyfip2 is needed for normal startle sensitivity. Finally, our data show that acute manipulation of the actin cytoskeleton substantially alters the startle threshold while also decreasing the number and size of excitatory synapses in inhibitory glycinergic neurons but not excitatory glutamatergic neurons. In Aim 3 we will test the hypothesis that cyfip2 acts cell-autonomously to maintain excitatory/inhibitory synaptic balance, combining behavioral recording with live imaging of neuronal activity and synaptic scaffolds to define direct links between cyfip2, circuit structure and function, and behavior. Overall, the results of this work will generate a detailed model of molecular and cellular pathways that control the startle behavior threshold and lay a foundation for understanding how these may be affected in human disease.
CHARGE syndrome is a rare, multi-system disorder that affects approximately 1 in 10,000 live births. The most common symptoms include ocular coloboma, choanal atresia, heart defects, genital abnormalities, ear malformations, and an array of neuro-behavioral difficulties. Sensory under- or over-load, motor impairments, enhanced pain, sleep disorders, attention deficit hyperactivity disorder (ADHD), obsessive compulsive disorder (OCD), intellectual disability, anxiety, and autism are all frequently observed in CHARGE. Treatments for these behavioral symptoms are limited, highlighting an area of critical need in understanding the mechanisms underlying these defects in order to develop new therapeutic approaches. Two-thirds of CHARGE cases are caused by loss-of-function mutations in chd7, which encodes a DNA-binding, ATP-dependent chromatin remodeling protein. chd7 is highly expressed in the developing vertebrate brain and regulates transcription of several key neurodevelopmental genes, but direct cellular and molecular links between chd7 function and behavioral regulation have not been established. This project leverages the larval zebrafish model, which has rapidly emerged as a powerful system for investigating the development and function of behavioral circuits as well as human genetic disease. Using CRISPR/Cas9 we have established a chd7-null line and have characterized several morphological and behavioral phenotypes reflective of CHARGE. In response to acoustic stimuli, chd7 mutants perform normal short-latency startle responses (SLCs) but have impaired long-latency escape responses (LLCs). Similarly, chd7 mutants respond normally to increases in illumination but are deficient in responding to decreases in illumination. These deficits are independent of morphological defects in the eyes and ears, indicating that chd7 likely regulates specific behavioral circuits in the brain. In Aim 1 we will systematically interrogate the known circuit elements driving these behaviors using in vivo calcium imaging and cell-specific rescue to locate the sites of chd7 action. We will then comprehensively define the brain regions that are dependent on chd7 using whole-brain morphometry and activity analyses. In Aim 2 we will apply state-ofthe- art proteomic and transcriptomic approaches to identify molecular pathways that link chd7 with these changes in brain structure and function. By analyzing samples from three developmental timepoints, we will also define the temporal dynamics of these changes. Finally, we will use a systematic CRISPR/Cas9 approach to validate the top proteomics- and transcriptomics-based chd7 targets in vivo by measuring brain development and behavior in mutant larvae. Overall, the results of this work will establish direct links between chd7, its molecular targets, and behavioral circuits. Furthermore, these aims will generate a powerful set of broadly useful resources for interrogating the cellular and molecular bases of chd7-dependent neural development.
Amyotrophic Lateral Sclerosis (ALS) is a progressive, fatal disease caused by degeneration of motor neurons in the brain and spinal cord. Although some genetic associations have been established, more than 90% of ALS cases are sporadic. Several environmental factors have been established as potential contributing factors, with exposure to cyanotoxins as a leading hypothesis. -N-methylamino-L-alanine (BMAA) is a neurotoxin produced by cyanobacteria around the world, particularly in fresh and marine water algal blooms that are exacerbated by the warming climate. BMAA has been linked to ALS-like disease since the 1940s and is hypothesized to exert its effects through neuronal excitotoxicity and/or misincorporation into brain proteins to produce misfolding, aggregates, and cell death. Several other cyanotoxins co-occur with BMAA in the environment, however, and in vitro data indicate they may interact synergistically to cause toxicity. Yet in vivo studies on the impact of cyanotoxin mixtures have not been done, and furthermore, in vivo data is needed to understand the cellular and molecular pathways that drive dysfunction to identify potential therapeutic targets. Here we propose to use a powerful vertebrate model system, the larval zebrafish, to investigate the toxicity of cyanotoxic mixtures in vivo. We will combine rigorous, high-throughput behavioral phenotyping of zebrafish larvae with confocal microscopy and state-of-the-art proteomic analysis to establish links between toxin mixture exposure and the underlying cellular and molecular perturbations driving ALS-associated pathology.
Behavioral issues pose a significant challenge for CHARGE syndrome patients and their caregivers. Although such issues vary widely, they commonly include attention deficit hyperactivity disorder (ADHD), obsessive-compulsive disorder (OCD), intellectual disability, sensory over- or under-load, diminished self-regulation, and anxiety. chd7, the gene mutated in most CHARGE syndrome cases, is highly expressed in the developing brain, but little is known about its role in neural development. Larval zebrafish, with their external development, large clutches of offspring, easy genetic manipulation, and diverse behavioral repertoire have emerged as a powerful vertebrate model system in which to study the development and activity of behavioral circuits. The goal of this project is to establish the larval zebrafish as a model in which to study the neural mechanisms underlying altered behavior in CHARGE syndrome. Preliminary data from CRISPR-injected mosaic chd7 mutant larvae demonstrate that they do recapitulate some behavior abnormalities associated with CHARGE syndrome including hyperactivity and repetitive movements during an open-field test. Using a high-throughput, unbiased behavioral analysis platform I propose to systematically test sensorimotor function and behavioral modulation in chd7 mutant zebrafish larvae. I also propose to take advantage of the zebrafish������������������s small size and optical clarity to identify brain regions that are affected by loss of chd7 using confocal microscopy to map neuronal activity across the entire brain. Data from these projects will provide a strong, essential foundation for future studies that will directly test the role of specific neurons and molecular pathways in the etiology of CHARGE-associated behavioral abnormalities.