Nick Buchler
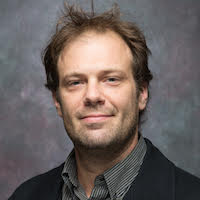
Bio
Dr. Nicolas Buchler joined NC State in July 2018 as a Chancellor’s Faculty Excellence Program cluster hire in Modeling the Living Embryo. His research is focused on understanding how the cell cycle interacts with metabolic rhythms, and how changes in these interactions lead to disease or new functions. The lab primarily works with early-diverging Fungi and yeasts, but they collaborate with others to study these same questions in other regulatory systems (e.g. circadian clocks) and organisms (e.g. animals and plants).
Dr. Buchler received a B.S. in Physics from the University of California at San Diego, before working on protein structure and evolution while obtaining a Ph.D. in Biophysics at the University of Michigan at Ann Arbor. During his post-doc, first at the University of California at San Diego and then at the Center for Studies in Physics & Biology at Rockefeller University, he combined mathematical modeling and experiments to understand how networks of genes sense and respond to multiple signals, store memories of past events, and schedule periodic events (e.g. cell cycle).
Education
PhD Biophysics University of Michigan 2001
Area(s) of Expertise
I am a biophysicist and geneticist by training with expertise in biological oscillations (e.g., cell cycle, metabolic rhythms), fungal genomics and evolution, single-cell gene expression, and mathematical modeling of gene regulatory dynamics.
Publications
- Deep learning-driven imaging of cell division and cell growth across an entire eukaryotic life cycle , MOLECULAR BIOLOGY OF THE CELL (2025)
- Deep learning-driven imaging of cell division and cell growth across an entire eukaryotic life cycle , (2024)
- Diploid-dominant life cycles characterize the early evolution of Fungi , PROCEEDINGS OF THE NATIONAL ACADEMY OF SCIENCES OF THE UNITED STATES OF AMERICA (2022)
- Gene expression noise accelerates the evolution of a biological oscillator , (2022)
- Competition for DNA binding between paralogous transcription factors determines their genomic occupancy and regulatory functions , GENOME RESEARCH (2021)
- Chytrid fungi. , Current biology : CB (2020)
- Genetic transformation of Spizellomyces punctatus, a resource for studying chytrid biology and evolutionary cell biology , eLife (2020)
- Enhancer Histone Acetylation Modulates Transcriptional Bursting Dynamics of Neuronal Activity-Inducible Genes , CELL REPORTS (2019)
- Epigenetic switching as a strategy for quick adaptation while attenuating biochemical noise , PLOS COMPUTATIONAL BIOLOGY (2019)
- Evolutionary innovation, fungal cell biology, and the lateral gene transfer of a viral KilA-N domain , CURRENT OPINION IN GENETICS & DEVELOPMENT (2019)
Grants
Metabolic rhythms occur in different cells and compartments within organisms. The origins and impact of these rhythms on other biological oscillators (e.g. cell cycle, circadian clocks) is only starting to be understood. The applicant������������������s long-term goal is to understand the mechanisms, function, and interaction of metabolic rhythms and the cell cycle in budding yeast, a model eukaryote. The yeast metabolic cycle (YMC) is a synchronous metabolic rhythm that occurs in a chemostat. The population synchrony arises from YMC-to-YMC coupling between cells via secreted metabolites. The YMC within each cell also interacts with the cell division cycle (CDC) to coordinate the events of carbon catabolism and cell cycle entry. These two oscillators have different periods, yet remain coordinated such that a fraction of the population commits to the CDC each YMC. Inference of YMC-CDC dynamics has been challenging because metabolic and cell cycle events are often measured and averaged across a heterogeneous population, which masks the dynamics that occur in a single cell. The objective of this proposal is to obtain new insights into these intracellular oscillators by measuring and perturbing the YMC and CDC in single cells. The central hypothesis is that the YMC and CDC can oscillate independently of one another but are normally coordinated in yeast through a reinforcing feedback loop (i.e., entry into a carbon catabolic state triggers the cell cycle, and, reciprocally, initiating the cell cycle triggers entry into a carbon catabolic state). The applicants will generate data that address the central hypothesis and its alternative with three specific aims: (1) Develop fluorescent reporter assays to measure population snapshots of metabolic and cell cycle states in single cells taken from a cycling chemostat; (2) Perturb the reinforcing feedback loop to disrupt the synchronization of YMC and CDC events in a cycling chemostat; and (3) Measure and perturb YMC-CDC dynamics outside the chemostat using timelapse fluorescence microscopy with microfluidics. Aim 1 will elucidate the timing and coordination of metabolic and cell cycle events in a cycling chemostat across different growth conditions. Aim 2 will directly test the reinforcing feedback loop that coordinates these oscillators in a cycling chemostat. Aim 3 will measure the extent to which metabolic rhythms occur in the absence of cell-to-cell communication via secreted metabolites and whether they remain coordinated with cell cycle events as seen in the chemostat. The observation that carbon catabolism and cell cycle entry remain coordinated in single cells across diverse growth conditions would strongly support the central hypotheses. This work is innovative because it combines single-cell technology and molecular genetics to address an unsolved problem in yeast with broad relevance to metabolic rhythms and cell cycle in other eukaryotes. This proposal is significant because it elucidates new mechanisms and regulatory principles of how intracellular oscillators with different frequencies can interact and remain functional.
Overview: Few processes in biology are as evolutionarily constrained as the cell division cycle, yet the eukaryotic cell cycle network in Fungi was rewired by a virus. Recent work from the PIs' laboratories showed that a horizontally-transferred viral protein (SBF) integrated into the G1/S regulatory network of a fungal ancestor and eventually replaced the ancestral transcription factor (E2F) in most modern Fungi without disrupting the cell cycle. Some early-diverging Fungi have a hybrid regulatory network with both SBF and E2F transcription factors, which is consistent with the hypothesized evolutionary transition state after the insertion of SBF and before the loss of E2F. Although these non-model organisms are poised to provide evolutionary insights, the G1/S cell cycle regulatory network in early-diverging Fungi is uncharted. The objective of this proposal is to use genomic methods to measure SBF and E2F DNA- binding specificity and the cis-regulatory architecture of G1/S cell cycle genes across early-diverging Fungi, with the goal of elucidating possible mechanisms by which an essential regulatory network was evolutionarily rewired by a virus. The central hypothesis is that the viral SBF initially gained cell cycle control by binding E2F-like cis-regulatory sequences of E2F-responsive promoters, and thereby gained the ability to control the G1/S transition. Early SBF then became redundantly integrated into the ancestral G1/S regulatory network, allowing the dikaryotic ancestor of Fungi to lose the E2F transcription factor. An alternative hypothesis is that SBF initially bound a different cis-regulatory sequence from E2F and regulated a different set of genes. Over time, evolution of cis-regulatory sites in many genes rendered SBF and E2F functionally redundant enough for E2F to be lost without disrupting the cell cycle. The applicants will generate data to address the central hypothesis and its alternatives with two specific aims: (1) Characterize the evolution of the DNA-binding specificity of recombinant SBF and E2F in early- diverging Fungi using high-throughput in vitro assays; and (2) Measure gene expression, chromatin accessibility, and transcription factor binding to promoters using genomic methods in cell cycle synchronized cultures of Spizellomyces punctatus (an early-diverging fungus known as a Chytrid). The in vitro data will identify overlapping and/or distinct DNA binding specificities and their conservation across early-diverging Fungi. The Spizellomyces data will identify cell cycle genes and elucidate the cis- regulatory architectures of SBF and E2F-regulated genes in an exemplary Chytrid that has both factors. Conservation of overlapping DNA-binding specificity of SBF and E2F across most early-diverging Fungi and redundant regulation of cell cycle genes in Spizellomyces would support the central hypothesis. This proposal is a first step towards the applicants' long-term goal of understanding how an essential cell cycle network was rewired by a viral protein without disrupting its function. Intellectual Merit: The proposed work is important because it addresses broad questions regarding cell cycle regulation (across Fungi and beyond), evolutionary acquisition and maintenance of essential pathways (here, the cell cycle network), and the potential role of viruses in the evolution of organisms. Developing Spizellomyces as a model organism for understanding cell cycle evolution should also help invigorate the study of Chytrids, which include the pathogenic Chytrid Batrachochytrium, the main cause of global diversity loss in amphibians. The applicants are well qualified for the proposed research because of their combined computational and experimental expertise in cell cycle systems biology, early-diverging Fungi, and regulatory genomics. Broader Impacts: Biology is becoming increasingly quantitative and technology-driven. The PIs will develop and teach a microbiology and microscopy high-school course. Time lapse movies easily capture the imagination and give students a more intuitive feel for b